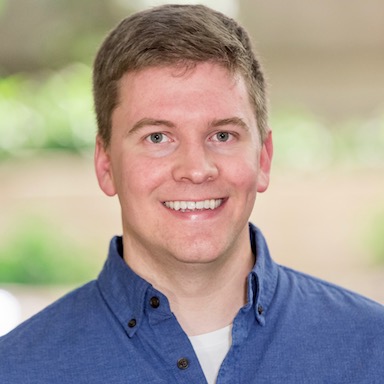
JD Emberson
JD is a computational cosmologist in the Argonne Computational Science (CPS) Division. He has a broad interest in the development and application of numerical techniques for large-scale cosmological structure formation simulations. This includes both N-body as well as hydrodynamic methods in order to gain insight into various astrophysical phenomena including dark energy, neutrinos, and primordial non-Gaussianity (see word cloud below). JD is one of the main developers of HACC with an emphasis on integrating robust hydrodynamical modeling for use on next-generation Exascale platforms. A large part of this effort includes understanding the systematic errors associated with the discrete nature of simulations and the various modeling choices made in multi-species and sub-resolution frameworks. JD has a keen interest in scientific data visualization; a collection of animations from his recent simulation endeavors can be found below.
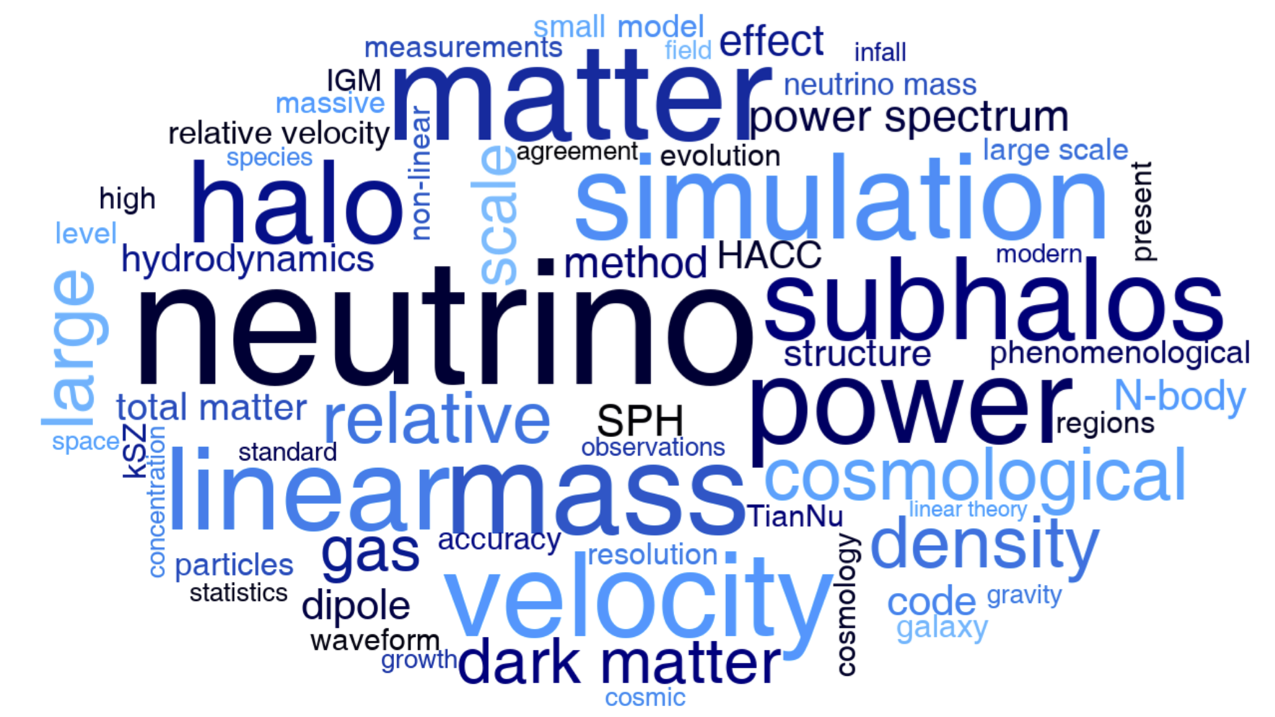
Codes
- SONICC is a python code that computes density and velocity transfer functions for use in the initial conditions of cosmological neutrino simulations. The transfer functions are backscaled from CAMB at a specified target redshift using a scale-dependent growth function that yields self-consistent evolution for all three matter species (cold dark matter, baryons, massive neutrinos) in the Newtonian forward model of the simulation.
Animations
Publications
- A Gigaparsec-Scale Hydrodynamic Volume Reconstructed with Deep Learning
C. Jacobus, S. Chabanier, P. Harrington, J. Emberson, Z. Lukić, and S. Habib
Submitted to ApJ. arXiv:2411.16920. - The picasso gas model: Painting intracluster gas on gravity-only simulations
F. Kéruzoré, L.E. Bleem, N. Frontiere, N. Krishnan, M. Buehlmann, J.D. Emberson, S. Habib, and P. Larsen
Accepted for publication in OJAp. arXiv:2408.17445. - Advances in ArborX to Support Exascale Applications
A. Prokopenko, D. Arndt, D. Lebrun-Grandie, B. Turcksin, N. Frontiere, J.D. Emberson, and M. Buehlmann
IJHPCA. DOI:10.1177/10943420241298296. arXiv:2409.10743. - Optimization and Quality Assessment of Baryon Pasting for Intracluster Gas using the Borg Cube Simulation
F. Kéruzoré, L.E. Bleem, M. Buehlmann, J.D. Emberson, N. Frontiere, S. Habib, K. Heitmann, and P. Larsen
OJAp, 6, 43 (2023). DOI:10.21105/astro.2306.13807. arXiv:2306.13807. - Numerical Discreteness Errors in Multi-Species Cosmological N-body Simulations
X. Liu, J.D. Emberson, M. Buehlmann, N. Frontiere, and S. Habib
MNRAS, 522, 3 (2023). DOI:10.1093/mnras/stad1176. arXiv:2303.00639. - Improving initialization and evolution accuracy of cosmological neutrino simulations
J.M. Sullivan, J.D. Emberson, S. Habib, and N. Frontiere
JCAP, 2023, 6, 3 (2023). DOI:10.1088/1475-7516/2023/06/003. arXiv:2302.09134. - Simulating Hydrodynamics in Cosmology with CRK-HACC
N. Frontiere, J.D. Emberson, M. Buehlmann, J. Adamo, S. Habib, K. Heitmann, and C.-A. Faucher-Giguere
ApJS, 264, 2 (2023). DOI:10.3847/1538-4365/aca58d. arXiv:2202.02840. - Modeling the Lyman-alpha forest with Eulerian and SPH hydrodynamical methods
S. Chabanier, J.D. Emberson, Z. Lukic, J. Pulido, S. Habib, E. Rangel, J. Sexton, N. Frontiere, and M. Buehlmann
MNRAS, 518, 3 (2023). DOI:10.1093/mnras/stac3294. arXiv:2207.05023. - Measuring the evolution of intergalactic gas from z=0 to 5 using the kinematic Sunyaev-Zel’dovich effect
J. Chaves-Montero, C. Hernandez-Monteagudo, R. Angulo, and J.D. Emberson
MNRAS, 503, 2 (2021). DOI:10.1093/mnras/staa3782. arXiv:1911.10690. - On the road to percent accuracy III: non-linear reaction of the matter power spectrum to massive neutrinos
M. Cataneo, J.D. Emberson, D. Inman, J. Harnois-Deraps, and C. Heymans
MNRAS, 491, 3 (2020). DOI:10.1093/mnras/stz3189. arXiv:1909.02561. - The Borg Cube Simulation: Cosmological Hydrodynamics with CRK-SPH
J.D. Emberson, N. Frontiere, S. Habib, K. Heitmann, P. Larsen, A. Pope, and H. Finkel
ApJ, 877, 85 (2019). DOI:10.3847/1538-4357/ab1b31. arXiv:1811.03593. - Cosmological neutrino simulations at extreme scale
J.D. Emberson, H.-R. Yu, D. Inman, T.-J. Zhang, U.-L. Pen, et al.
RAA, 17, 085 (2017). DOI:10.1088/1674-4527/17/8/85. arXiv:1611.01545. - Measurement of the Cold Dark Matter-Neutrino Dipole in the TianNu Simulation
D. Inman, H.-R. Yu, H.-M. Zhu, J.D. Emberson, et al.
Physical Review D, 95, 083518 (2017). DOI:10.1103/PhysRevD.95.083518. arXiv:1610.09354. - Differential Neutrino Condensation onto Cosmic Structure
H.-R. Yu, J.D. Emberson, D. Inman, T.-J. Zhang, U.-L. Pen, et al.
Nature Astronomy, 1, 0143 (2017). DOI:10.1038/s41550-017-0143. arXiv:1609.08968. - Evolution of Low Mass Galactic Subhalos and Dependence on Concentration
J.D. Emberson, T. Kobayashi, and M.A. Alvarez
ApJ, 812, 9 (2015). DOI:10.1088/0004-637X/812/1/9. arXiv:1504.00667. - Precision reconstruction of the dark matter-neutrino relative velocity from N-body simulations
D. Inman, J.D. Emberson, U.-L. Pen, A. Farchi, H.-R. Yu, and J. Harnois-Deraps
Physical Review D, 92, 023502 (2015). DOI:10.1103/PhysRevD.92.023502. arXiv:1503.07480. - High Performance P3M N-body code: CUBEP3M
J. Harnois-Deraps, U.-L. Pen, I.T. Iliev, H. Merz, J.D. Emberson, and V. Desjacques
MNRAS, 436, 540 (2013). DOI:10.1093/mnras/stt1591. arXiv:1208.5098. - The Opacity of the Intergalactic Medium During Reionization: Resolving Small-Scale Structure
J.D. Emberson, R.M. Thomas, and M.A. Alvarez
ApJ, 763, 146 (2013). DOI:10.1088/0004-637X/763/2/146. arXiv:1208.3679. - Interpolation in waveform space: enhancing the accuracy of gravitational waveform families using numerical relativity
K. Cannon, J.D. Emberson, C. Hanna, D. Keppel, and H. Pfeiffer
Physical Review D, 87, 044008 (2013). DOI:10.1103/PhysRevD.87.044008. arXiv:1211.7095.